Kinetic Studies of Nuclear Import Pathways
We are also dissecting the NPC architecture of a highly divergent eukaryote, Trypanosoma brucei in order comparing it to the available structures from yeast and humans, to unravel the molecular processes that governed the evolution and re-purposing of membrane-coating complexes during the origin of the nucleus, and to provide orthogonal insights into what are the most essential features of the nucleocytoplasmic transport machinery. Moreover, we are studying NPCs in human cells to determine how alterations in the NPC during oncogenesis and viral infection can lead to disease.
We are studying the kinetics and interactions between transport factors, transport cargoes, and NPC. Rather than a single import/export cycle (as once envisioned), many different but partially redundant and overlapping transport pathways all converge at the NPC, and the design of the NPC must reflect this. On the basis of our work, we also proposed the “virtual gating” hypothesis to explain how the NPC mediates these multiple gated nuclear transport pathways: the NPC can be likened to a catalyst, where diffusion of macromolecules is largely prevented by and entropic barrier which can only be overcome by the binding energy (i.e. enthalpy) of specific transport factors, thus facilitating the exchange of transport factor-cargo complexes across the NE.
Our eventual aim is to integrate our ultrastructural and biochemical studies to understand the molecular basis of the translocation of different transport factors across the NPC. These studies should enable us to reconstitute key reactions of these processes in vitro, study the high-resolution structures mediating the transport processes and test possible mechanistic models in vivo to understand the complete sequence of events during a transport cycle.
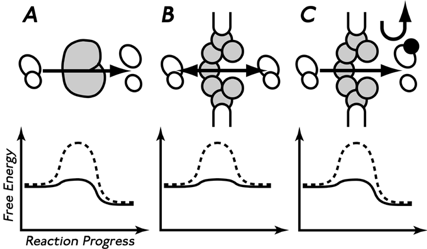
Virtual gating: the NPC is analogous to an enzyme.
(A, top), a classical enzyme (gray) converting a substrate (left) to products (right).
(A, bottom) graph showing the energy of reactants and products, and the activation energy, with (solid line) and without (dashed line) the enzyme.
(B), the NPC (gray) catalyzing diffusion across the NE.
(C), RanGTP binding providing directionality (import) via its chemical gradient across the NE.
The NPC contains particular components termed FG Nups, so called because they carry intrinsically disordered regions that contain multiple phenylalanine–glycine (FG) repeats; their interactions with transport factors (TFs) underlie the paradoxically rapid yet also highly selective transport of macromolecules mediated by the nuclear pore complex (NPC), by forming a dynamic FG-repeat phase through which cargo-carrying transport factors readily pass, facilitated by their specific FG interactions, whereas nonspecific macromolecular diffusion is hindered by this same dense phase. Several models have been proposed to explain at the molecular level how FG repeat regions can form a barrier to the passage of most molecules, but these could not fully explain how FG Nups could do this while simultaneously allowing the selective and fast transport of nuclear transport receptors.
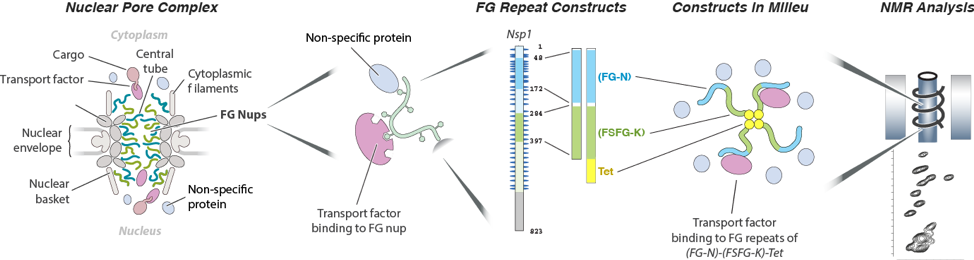
NMR Analyses of FG repeats.
Our experimental approach includes key features of the NPC; a mixture of FG flavors, attachment at one end, and both specific (TF) and non-specific interactions with the cellular milieu. For example, our largest construct (FG-N-FSFG-K-tet) contains two FG flavors (FG-N, turquoise; FSFG-K, green; full length Nsp1 also shown with residue numbering), a separator (white) and the tetramerization domain of p53 (yellow). NMR analysis is performed on this construct and its variants, in cell-mimicking milieu of various types; changes in position or intensity of peaks (bottom right) indicate changes in structure or interactions of the FG motifs.
Because there has also been a major lack of experimental data that probed the behavior of FG nucleoporins in detail, we applied nuclear magnetic resonance spectroscopy (NMR) to analyze the dynamic behavior and interactions of the FG repeat regions at the atomic scale. This revealed that FG nucleoporins never adopt an ordered three-dimensional shape, even briefly; instead they are true intrinsically disordered proteins, remaining unfolded and disordered and in constant motion. We were also able to detect that FG repeats engage briefly with a series of pockets present on the transport factors. Kd values between the interacting and non-interacting ensembles for increased numbers and spacing of motifs show a local concentration effect with very modest avidity, and fast exchange.
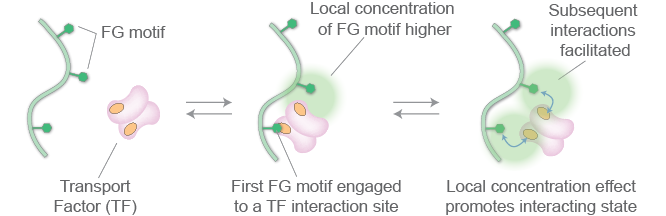
Schematic diagram of the local concentration effect promoting the ‘interacting state’.
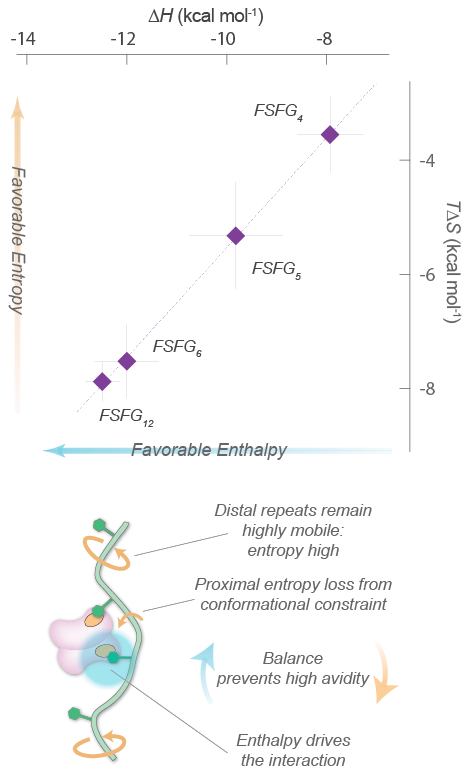
Thermodynamics of FG repeat – transport factor interactions.
Top, Enthalpy-entropy compensation curve for the interactions between constructs with different numbers of FG repeats (FSFG4-FSFG12) and the transport factor NTF2, with a linear fit. Bottom, Schematic diagram of the enthalpy-entropy balance that prevents high avidity interactions.
These FG repeats can bind and then release the transport factors’ binding pockets at unusually high speeds, which enables the transport factors to move quickly through the nuclear pore complex. This transit is specific because only transport factors have a high capacity for interacting with the FG repeats. By combining NMR with isothermal titration calorimetry (ITC) to thermodynamically characterize these multivalent interactions, we revealed that a combination of low per-FG motif affinity and the enthalpy–entropy balance proposed by our virtual gating model prevents high-avidity interaction between FG Nups and TFs, whereas the large number of FG motifs promotes frequent FG–TF contacts, resulting in enhanced selectivity. These findings underscore the importance of functional disorder of FG Nups, and help explain the rapid and selective translocation of TFs through the nuclear pore complex. This work also further expands our understanding of the functional roles of “fuzzy” interactions involving intrinsically disordered proteins, which make up a major class of macromolecules in eukaryotes.
Our eventual aim is to integrate our ultrastructural and biochemical studies to understand the molecular basis of the translocation of different transport factors across the NPC. These studies should enable us to reconstitute key reactions of these processes in vitro, study the high-resolution structures mediating the transport processes and test possible mechanistic models in vivo to understand the complete sequence of events during a transport cycle.
A Few Nanoseconds Simulation of Kap – FG Interactions
References
-
L. Timney, B. Raveh, R. Mironska, J.M. Trivedi, S.J. Kim, D. Russel, S.R. Wente, A. Sali, M.P. Rout. Simple rules for passive diffusion through the nuclear pore complex. J Cell Biol. 2016 Oct 10;215(1):57-76. PMCID: PMC5057280
-
E. Hough, K. Dutta, S. Sparks, D.B. Temel, A. Kamal, J. Tetenbaum-Novatt, M.P. Rout, D. Cowburn. The molecular mechanism of nuclear transport revealed by atomic scale measurements. Elife. 2015 Sep 15;4. pii: e10027. doi: 10.7554/eLife.10027. PMCID: PMC4621360
-
Jovanovic-Talisman T, Tetenbaum-Novatt J, McKenney AS, Zilman A, Peters R, Rout MP, Chait BT. Artificial nanopores that mimic the transport selectivity of the nuclear pore complex. Nature. 2009 Feb 19;457(7232):1023-7. PMCID: PMC2764719
-
Hayama, S. Sparks, L.M. Hecht, K. Dutta, C.M. Cabana, J.M. Karp, M.P. Rout*, D. Cowburn*. Thermodynamic characterization of the multivalent interactions underlying rapid and selective translocation through the nuclear pore complex. J Biol Chem. 2018 Mar 23;293(12):4555-4563. doi: 10.1074/jbc.AC117.001649. Epub 2018 Jan 26. PMCID: PMC5868264 (Featured: Editor’s Pick)